Cardiac Function and Monitoring
Cardiac Function
- Determinants of Cardiac Output (CO)
- CO = SV x HR
- SV = EDV - ESV
- SV is a function of 3 variables: preload, afterload, and contractility
- normal stroke volume is 50 – 100 ml
- ejection fraction (EF) = ESV / EDV
- normal EF is 0.5 – 0.7
- Preload
- defined as the force acting on a muscle just before contraction
- as preload increases, muscle length increases and tension during contraction increases to a
maximum and then declines as stretching continues
- Frank-Starling Law of the Heart: the energy of contraction is proportional to the initial
length of cardiac muscle fiber
- in the heart, the initial length of the muscle fiber before contraction is a function of the
end-diastolic volume
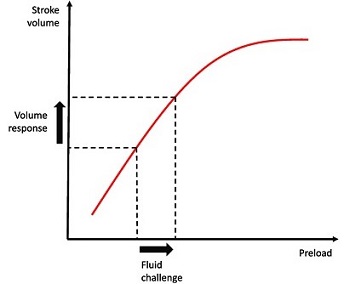
- Determinants of Preload
- most important determinant of preload is intravascular volume
- venous return is also influenced by venous capacitance (tone), which in turn is
influenced by many drugs (morphine, nitroglycerine, catecholamines)
- increased intrathoracic pressure from positive-pressure ventilation and PEEP will
decrease venous return (and preload) as well
- Afterload
- defined as the tension that a muscle must develop before it can begin to shorten
- in the heart, afterload is the arterial resistance against which the heart must overcome to
eject blood into the systemic circulation (systemic vascular resistance)
- systemic vascular resistance: SVR = 80 x (MAP – CVP) / CO
- an increase in afterload will increase the amount of energy required for contraction
- as more work goes into raising intraventricular pressure above the SVR, less work will be
available for the ejection of blood
- an increase in afterload results in a decrease in stroke volume and vice versa
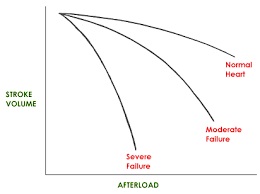
- Myocardial Contractility
- defined as the inotropic state of the myocardium
- contractility increases when the force of ventricular contraction increases when preload
and afterload are held constant
- inotropic state of the heart can be influenced by endogenous and exogenous catecholamines
- ejection fraction is a useful indicator of ventricular function, but it is difficult to
measure clinically
- Heart Rate and Rhythm
- increases in heart rate are directly related to increases in cardiac output
- however, if the heart rate is too high, the diastolic interval is short enough to interfere
with ventricular filling
- cardiac rhythm is also important to cardiac performance
- in sinus rhythm there is a coordinated depolarization of the atria and ventricles, which
improves ventricular filling and cardiac output
- in atrial fibrillation, loss of the ‘atrial kick’ can result in a significant decline in
ventricular filling and cardiac output
- Compliance
- relationship between filling pressure and volume: C = ΔV / ΔP
- as compliance decreases, the ventricle becomes stiffer and less distensible
- this may produce less diastolic filling and decreased cardiac output
- myocardial ischemia, hypertrophy, restrictive cardiomyopathies, pericarditis, and
pericardial tamponade decrease ventricular compliance
Cardiovascular Monitoring
- Central Venous Pressure Monitoring
- right atrial pressure monitoring is a more accurate term
- right atrial pressure is a close approximation of the right ventricular end-diastolic volume and
reflects the adequacy of intravascular volume (preload)
- tricuspid valve, intrathoracic pressure, and right ventricular compliance must be normal for the
measurements to be valid
- Pulmonary Artery Catheters
- Indications
- provides a number of easily measured or calculated hemodynamic parameters: right atrial
(CVP) pressure, pulmonary artery pressures, pulmonary capillary wedge pressure, cardiac
output, mixed venous oxygen saturation, and systemic vascular resistance
- information obtained from pulmonary artery catheterization allows rational decisions to be
made about therapies, and the impact of these therapies can be measured
- however, there is scant evidence that pulmonary artery catheters lead to better patient
survival
- a 1996 study concluded that placement of a pulmonary artery catheter within the first 24 hours of
stay in an ICU was associated with a significant increase in the risk of mortality
- lack of effectiveness of PA catheters may be due to several reasons: 1) morbidity/mortality
of the catheter itself; 2) data generated may be inaccurate; 3) even accurate data may be misinterpreted
- nevertheless, more precise knowledge of fundamental cardiovascular parameters can facilitate
earlier diagnosis and guide therapy
- conditions that may benefit from placement of a pulmonary artery catheter include oliguria
despite adequate fluid resuscitation, severe burns or multisystem trauma, postoperative
cardiovascular complications (myocardial infarction, pulmonary edema, CHF),
differentiating between ARDS and pulmonary edema, and assessing the effects of high levels
of PEEP on cardiac function
- Risks
- arrhythmias, including complete heart block if the patient has a pre-existing left bundle
branch block
- coiling or knotting of the catheter
- pulmonary artery rupture and hemorrhage
- valve damage, endocarditis
- catheter fracture, balloon rupture
- catheter-related sepsis, pneumothorax
- Clinical Utility
- Pulmonary Artery Wedge Pressure
- the true goal of fluid management is to achieve optimal loading of the left ventricle
- best measure of left-sided filling is the LVEDV
- left atrial pressure is the best approximation of LVEDP
- LVEDP reflects LVEDV as long as there is no alteration in left ventricular compliance
- to directly measure left heart pressures requires cardiac catheterization
- pulmonary artery wedge pressure (PCWP) is a very accurate indicator of left atrial
pressure, and assuming a normal mitral valve, an accurate indicator of LVEDP
- once the catheter reaches the pulmonary artery, the balloon is inflated and advanced
until it wedges in a small branch of the pulmonary artery
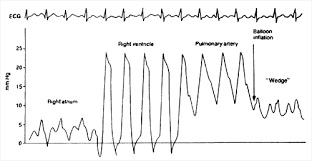
- Pitfalls
- if the catheter is positioned in areas of the lung where alveolar pressure
exceeds pulmonary venous pressure (zones 1 and 2), then the PCWP may reflect
alveolar pressure and not left atrial pressure
- fortunately, since the PA catheter is flow-directed, it is most likely to
pass into areas of the lung where flow is high and both pulmonary artery and
venous pressures exceed alveolar pressure
- high levels of PEEP (> 10 cm water) can spuriously raise the PCWP by raising
intrathoracic vascular pressure
- Cardiac Output
- measured by the thermodilution technique
- accuracy only ± 15%
- known volume of cold saline is injected into the right atrium
- the change in temperature is measured by a thermistor located in the pulmonary artery
- the greater the magnitude of the temperature drop in the pulmonary artery, the lower
the rate of flow, and vice versa
- in actuality, right ventricular output is being measured, which is equivalent to
left ventricular output unless there is intracardiac shunting
- technical errors that decrease accuracy include the slow or erratic injection of the
indicator, inaccurate measurement of the volume and temperature of the indicator,
improper placement of the catheter
- 3 measurements should be averaged, since a single measurement is unreliable
- Calculated Hemodynamic Values
- most commonly used calculated value is the systemic vascular resistance (SVR)
- additional calculated values that may be useful in critically ill patients include
arterial blood oxygen content (CaO2), mixed venous blood oxygen content (CvO2), and
oxygen consumption (VO2)
Alternatives to the Pulmonary Artery Catheter
- Assessing Volume Status and Fluid Responsiveness
- Pulse Pressure Variation (PPV)
- pulse pressure is the difference between systolic and diastolic arterial blood pressures
- pulse pressure varies with expiration and inspiration during positive pressure ventilation
- pulse pressure variation is a marker of the position on the Frank-Starling curve, and is
not a measure of preload
- a PPV of > 13% - 15% is strongly associated with volume responsiveness
- a number of commercially available monitors that can measure PPV exist
- technique is limited to mechanically ventilated patients in sinus rhythm who are receiving a
low tidal volume and who are not spontaneously triggering the ventilator
- Passive Leg Raising
- provocative maneuver designed to assess whether or not a patient is fluid responsive by
autotransfusing blood from the legs into the chest
- must be combined with a way to measure cardiac output or PPV
- the patient is positioned with the head and torso elevated to 45 degrees, and a baseline
measurement of cardiac output is obtained
- the patient is then rapidly positioned with their head and torso flat, and the legs are
raised and held at 45 degrees for one minute
- another measure of cardiac output is obtained
- a 10% increase in cardiac output predicts fluid responsiveness
- Vena Cava Assessment
- vena cava diameter and dynamic measures of vena cava collapse have been proposed as tools
for estimating intravascular volume status
- since there are no valves between the vena cava and the right atrium, fullness of the vena
cava is thought to correlate with right atrial pressure
- the diameter of the IVC will change with respiration
- in a spontaneously breathing patient, a decrease in intrathoracic pressure with inspiration
will lead to collapse of the IVC
- in a mechanically ventilated patient, increased intrathoracic pressure will cause distention
of the IVC
- a change in IVC diameter with respiration of 12% - 18% has been associated with fluid
responsiveness
- Measuring Cardiac Output
- Arterial Waveform Analysis
- uses the arterial pressure waveform as an input for a model of the systemic circulation
- other parameters must be estimated initially: resistance, compliance, impedance
- Aortic Doppler
- used to measure RBC velocity in the aorta, from which blood flow rate can be calculated
- the probe may be placed in the esophagus (in an intubated and sedated patient) or on the
anterior chest
- major limitation of this technique is correct positioning of the probe, as it must be well
aligned with the direction of blood flow
- Transesophageal Echocardiography
- patient must be sedated and intubated for airway protection
- a small probe can be left in place for 72 hours
- LV and RV function can be assessed
- ventricular volume, ejection fraction, and cardiac output can be determined
- technique requires considerable skill and training to produce reliable results
Pharmacologic Interventions
- Adrenergic Receptors
- alpha-1
- located primarily on vascular smooth muscle
- stimulation results in contraction
- beta-1
- found predominantly in the heart
- stimulation results in ↑ contractility, ↑ heart rate, ↑ conduction velocity
- beta-2
- found predominantly in smooth muscle
- stimulation results in relaxation of bronchial, uterine, gastrointestinal smooth muscle
- Dopaminergic Receptors
- found in renal, mesenteric, cardiac, and smooth muscle vascular beds
- effects are dose- and organ-dependent
- Vasopressin Receptors
- V1
- located on vascular smooth muscle
- stimulation causes vasoconstriction
- V2
- located on distal convoluted tubule and collecting duct of the kidney
- stimulation causes water retention
- Inotropic Agents
- Epinephrine
- adrenal medullary hormone
- stimulates both α + β receptors
- in volume-loaded patients, increases heart rate, cardiac output, stroke volume, and systemic
oxygen delivery without affecting PCWP or SVR
- Norepinephrine
- immediate precursor to epinephrine
- neurotransmitter
- potent α1 agonist (vasoconstriction)
- minimal β2 effects
- Dopamine
- immediate precursor to norepinephrine
- effects mediated by 3 different receptors, depending on the dose
- at 1 to 4 μg/kg/min, produces renal and mesenteric vasodilation via dopamine receptors
- at 5 to 10 μg/kg/min, increases heart rate and contractility through stimulation of
β1 receptors
- at > 10 μg/kg/min, has primarily α1 effects
- at high doses renal vasodilation is lost
- undesirable effects include tachycardia, arrhythmias, increased systemic oxygen demand,
increased PCWP
- Dobutamine
- synthetic amine
- predominantly a β receptor agonist
- β1 effects lead to increased myocardial contractility and heart rate
- β2 effects lead to decreased SVR and increased myocardial blood flow
- powerful inotropic agent; weak chronotropic agent
- Milrinone
- phosphodiesterase inhibitor
- causes increase in cardiac contractility and vasodilation in systemic and pulmonary beds
- long half-life is a disadvantage
- excessive vasodilation often requires norepinephrine support
- works synergistically with dobutamine to increase cardiac contractility
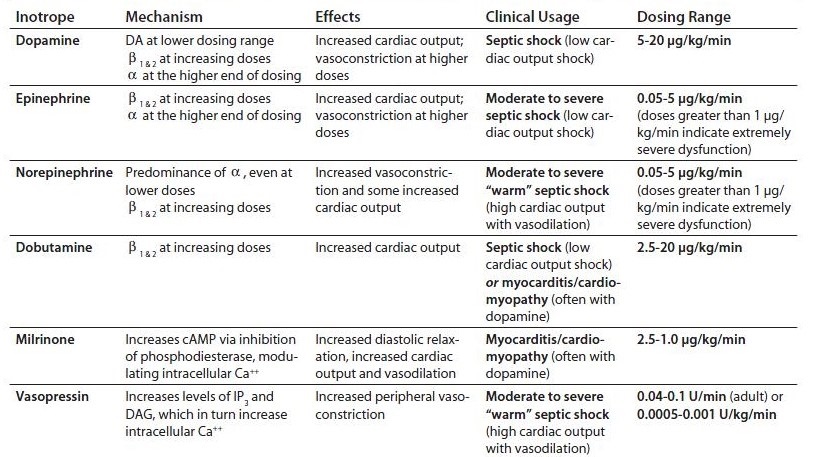
- Vasodilators
- Nitroprusside
- smooth muscle vasodilator
- affects all vascular beds: arterial, venous, and coronary
- effective afterload reducing agent
- metabolized to cyanide
- cyanide toxicity is rare when the drug is used for less than 48 hours
- Nitroglycerin
- smooth muscle vasodilator
- at low doses is primarily a venodilatory agent
- at higher doses is an arterial vasodilator
- at lower doses is also a coronary artery vasodilator
- reduces preload, which reduces myocardial oxygen consumption
- tachyphylaxis may develop over time
- Calcium Channel Blockers
- have a negative inotropic effect
- produce vasodilation by interfering with calcium-induced smooth muscle contraction
- prolong conduction through the AV node
- Angiotensin-Converting Enzyme Inhibitors
- interferes with the pulmonary conversion of angiotensin I to angiotensin II
- produce a significant reduction in SVR by producing vasodilation and diminishing plasma
volume
Mechanical Assist Devices
- Intraaortic Balloon Pump (IABP)
- supports the circulation by 2 mechanisms:
- rapid inflation of the balloon during diastole causes ↑ diastolic pressure and ↑ coronary
and peripheral perfusion
- deflation before systole results in ↓ afterload and improved myocardial performance
- Left Ventricular Assist Devices
- devices that pump blood from the left ventricle or left atrium to the aorta
- may be placed percutaneously through the femoral artery or surgically implanted
- have not been shown to improve mortality
References
- Schwartz, 10th ed., pgs 399 - 409
- O’Leary, pgs 360 - 370
- Simmons and Steed, pgs 193 – 202
- Mattox, 4th ed., pgs 1287 - 1296
- UpToDate. Novel Tools for Hemodynamic Monitoring in critically Ill Patients with Shock.
Mark E. Mikkelsen, MD, MSCE, David F. Gaieski, MD, Nicholas J. Johnson, MD. Sep 28, 2020, pgs 1 – 30.