DNA Synthesis and Transcription
- Chromosomes
- interior of the nucleus contains 23 paired chromosomes carrying 25,000 - 30,000 genes
- each chromosome is composed of nearly equal amounts of deoxyribonucleic acid (DNA) and basic histone proteins
- DNA component is composed of 2 deoxyribonucleotide polymers coiled into a double helix
- the basic repeating unit of each polymer is a deoxyribose sugar with a phosphate attached at one end and
a nitrogenous base attached to the other
- the two nucleotide chains are held together by hydrogen bonds which join the purine bases adenine (A) and
guanine (G) with the pyrimidine bases thymine (T) and cytosine (C)
- base pairing is invariant: every A is paired to a T; every G is paired to a C
- hence, the base sequence of one chain exactly specifies the base sequence of the other chain
(the chains are complementary)
- DNA must be tightly packed to fit inside the nucleus - histone proteins are instrumental in this function
- the telomere is a specialized DNA cap at the end of the chromosome - its major function appears to be prevention
of chromosomal shortening during replication
- DNA Replication
- DNA Synthesis
- DNA is duplicated by the unwinding and use of each chain as a template for copying by complementary base pairing
- this results in semiconservative replication - each DNA molecule is composed of one old (conserved) and one new chain
- DNA polymerase catalyzes nucleotides into DNA
- in addition to polymerizing DNA, DNA polymerase is also responsible for editing and proofreading, gap filling,
and repair functions
- two complete DNA sequences are formed that contain identical genetic information
- a mistake in DNA synthesis is called a mutation, and will result in incorrect DNA sequences being copied to daughter cells
- a mistake in a single base pair is called a point mutation, potentially leading to either a missense or nonsense mutation
- missense mutations can result in a single amino acid being changed, which can lead to alterations in protein structure
and function
- nonsense mutations result in replacement of an amino acid with a stop codon, leading to premature termination of
protein translation
- frameshift mutations result from the addition or deletion of several amino acids, leading to the insertion of an unrelated
amino acid or stop codon
- some mutations are silent and do not affect function of the protein
- Cell Cycle
- cells spend most of their time in the G0 state
- cells stimulated to divide enter the cell cycle: G1==>S==>G2==>M
- S phase is where DNA synthesis occurs
- M phase (mitosis) is where the nucleus and cell divide
- the cell cycle is tightly regulated to ensure that cells divide only when necessary
- one of the hallmarks of cancer is the loss of cell cycle regulation
- Cell Cycle Control
- progression through the cell cycle phases is governed by the sequential
activation and inactivation of a family of regulatory proteins called cyclin-dependent kinases (CDKs)
- CDK activation requires the binding of a regulatory protein (cyclin)
- CDK activity is inhibited by CDK inhibitory proteins (CKIs)
- the active cyclin-CDK complex phosphorylates other cell cycle regulatory proteins
- multiple cyclin/cyclin-dependent kinase complexes exist and exhibit a cell cycle phase specificity
- mutations that cause increased activity of the cyclin/CDK complexes are important factors for malignant transformation
- p53
- transcription factor that binds to DNA, activating transcription of p21
- p21 blocks the activity of a cyclin-dependent kinase required for progression through G1
- this block allows time for the cell to repair any DNA damage before it is replicated
- if the genomic damage cannot be repaired, then the progression through the cell cycle is
stopped and apoptosis induced
- functions as a tumor suppressor and is the most frequently mutated gene in human cancers
- RNA Transcription
- RNA Synthesis
- nucleolus is the site where most cellular RNA is produced and organized
- mRNA contains the base sequence that codes for the amino acids of the newly synthesized protein
- uracil (U) is substituted for thymine, such that U pairs with A as its complementary base
- RNA transcription is asymmetric because RNA polymerase selectively copies only one of the
two DNA strands – the choice of strand is dependent on the gene
- mRNA synthesis first involves a large precursor molecule that contains both protein coding
sequences (exons) and intervening noncoding sequences (introns)
- the final mRNA molecule is shortened by splicing to contain only exons
- Control of RNA Synthesis
- transcription is highly regulated
- RNA synthesis begins with binding of RNA polymerase to an upstream promoter region (TATA box)
- there are thousands of gene regulatory proteins – transcription factors -, which bind to
specific DNA sequences called regulatory elements
- transcription factors activate or repress transcription, and different regulatory proteins are
expressed in different cells
- many human genes have more than 20 regulatory elements
- one example of a transcription factor is the steroid hormone receptor-ligand complex
- RNA Translation
- synthesis of proteins from mRNA
- takes place on ribosomes in the cytoplasm
- a codon, which is a triplet of three mRNA bases, encodes for one amino acid
- each codon is recognized by a tRNA molecule, which adds the correct amino acid to the growing peptide chain
- most amino acids are coded by more than one codon
- the start codon is AUG; there are three stop codons
- most proteins are modified by some combination of proteolytic cleavage, glycosylation, phosphorylation, and sulfation
- many of the modifications occur in the Golgi apparatus
Cell-to-Cell Interactions
- multi-celled organisms have evolved an elaborate system of cell-to-cell communication
- extracellular information that cells process is provided in the form of various humoral or contact-mediated signals
- these signals (ligands) exert their effects by binding with specific transmembrane receptor proteins
- this is then transduced into a cytoplasmic signal, which initiates a second messenger cascade within the cell
- Chemical Signaling
- various strategies have evolved to transport information to cells at some distance
- Endocrine Signaling
- long-range signaling requires the transport of signals via the bloodstream
- specific messenger molecules (hormones) are secreted by specialized cells which are usually organized
into glands
- endocrine signals reach every cell in the body, but only those cells that are able to bind the
signal and translate its message will respond
- Paracrine Signaling
- paracrine signals act over short distances
- typical examples are cytokines, eicosanoids, and biologically active amines such as serotonin or histamine
- do not normally enter the circulation in sufficient concentration to affect distant cells
- various control mechanisms normally keep paracrine signals confined to their immediate region
(proteinases, soluble receptors)
- spillover of paracrine signaling molecules can have profound systemic effects (tumor necrosis factor, IL-1)
- Autocrine Signaling
- autocrine signals act on the secreting cell itself, provided that the cell can receive its own signals
- this provides feedback regulation of cell function
- Synaptic Signaling
- nerve cells transmit information by electrical excitation across long distances to well defined target cells
- target cells are contacted through synaptic signaling by the release of specific neurotransmitters
- Receptors
- whether the route of signaling follows an endocrine, paracrine, autocrine, or synaptic fashion, the ligand will bind
to a protein receptor that translates the signal into information that leads to a specific reaction of the
target cell
- water-soluble ligands (proteins) bind to cell surface receptors
- lipid-soluble ligands can cross biologic membranes and bind to receptors in the cytoplasm or nucleus
- Intracellular Receptors
- thyroid and steroid hormones pass directly through the plasma membrane and bind to receptor proteins inside the cell
- resulting complex is then transported into the nucleus
- hormone-receptor complex then binds to certain regions of the DNA, regulating transcription of various genes
- Cell Surface Receptors
- binding of ligands to cell surface receptors initiates a cascade of intracellular second messenger systems that
regulate cell function directly or change gene expression
- Tyrosine Kinase Receptors
- translate specific signals into tyrosine kinase activity
- controls cellular events by phosphorylation of tyrosine residues located in certain intracellular proteins
- this causes a conformational change sufficient to initiate a specific intracellular response
- receptor family includes epidermal growth factor (EGF), platelet-derived growth factor, transforming growth factor-α,
insulin receptor
- erb B oncogene encodes for a mutation of the receptor for EGF
- G Protein-linked Receptors
- G proteins function as mediators between G protein-linked receptors and membrane-associated enzymes
- G proteins can function as stimulators or inhibitors of signal transduction
- binding of the ligand to its receptor changes the configuration of the receptor, which enables binding of
the G protein to the receptor
- GDP of the G protein is then replaced by GTP
- G protein then dissociates from its receptor
- G protein can now bind to and activate specific membrane-bound enzymes
- oncogenes such as the ras proteins are involved in G protein-coupled signal transduction
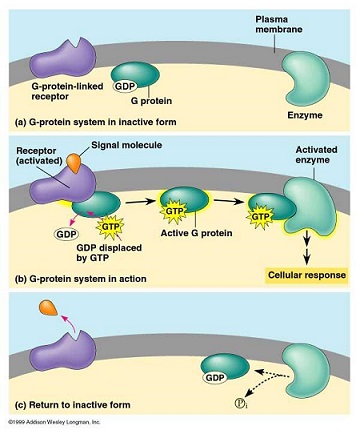
- Intracellular Processing of Signals
- following receptor-ligand interactions, events occurring in cell membranes must be translated into messages that can be
interpreted within the cell
- this is organized through stimulation of second messenger systems
- cAMP
- ubiquitous intracellular messenger in all animal cells
- produced by adenylate cyclase using ATP
- activity of adenylate cyclase is regulated by G protein-coupled cell surface receptors
- 2nd messenger function of cAMP is mediated through activation of cAMP-dependent protein kinases
- these enzymes in turn activate other enzymes by phosphorylation
Cell Death
- to maintain tissue homeostasis, cell proliferation must be balanced against cell death
- cell death is responsible for removing senescent cells and cells with genetic damage beyond repair
- Apoptosis
- programmed cell death is activated by two pathways, the extrinsic pathway, and the intrinsic pathway
- in the extrinsic pathway, cell surface death receptors bind to proapoptotic ligands (TNF)
- the intrinsic pathway is activated when intracellular sensors (p53) detect proapoptotic stimuli
such as irreparable genetic damage
- both activated pathways result in activation of caspases (cysteine aspartase proteases) that cleave
proteins after aspartic acid residues
- activated caspases set off a terminal series of events by cleaving key cellular proteins – nuclear
and cytoskeletal structural proteins, DNA repair proteins
- process results in formation of vesicles called apoptotic bodies, which are then eliminated by phagocytic cells
- dysregulation of apoptosis can lead to cancer or autoimmune diseases
- Autophagy
- characterized by massive vacuolization of the cytoplasm without chromatin condensation
- resulting vesicles are called autophagosomes
- autophagosomes fuse with lysosomes, leading to degradation of engulfed cytoplasmic material and organelles
- plays an important role in protecting against infection, neurodegeneration, and tumor development
- autophagy is controlled by at least 11 highly conserved genes
- process is less well understood than apoptosis
References
- Simmons and Steed, pgs 3 - 12
- O’leary, 4th ed., pgs 1 – 43
- Sabiston, 19th ed., pgs 24 - 39
- Schwartz, 10th ed., pgs 443 - 453